
The 2008 Nobel Prize in Physics has been awarded to three men whose work has contributed significantly to our understanding of why we're here. Makoto Kobayashi of the High Energy Accelerator Research Organisation, Japan, and Toshihide Maskawa of Kyoto Sangyo University share one half of the prize, with the remaining half going to Yoichiro Nambu of the University of Chicago. Their combined body of work paves the way towards solving two of the biggest mysteries of physics.
![]() Makoto Kobayashi. Photo: KEK |
![]() Toshihide Maskawa. Photo: Kyoto University |
![]() Yoishiro Nambu. Photo: University of Chicago |
Where are our anti-twins?
Physicists believe that the Universe erupted into being around 14 billion years ago, when the Big Bang caused its initial extremely hot and dense state to expand and cool. Within split seconds after the Big Bang the fundamental particles that make up all matter we see in the Universe today and the fundamental forces that govern their interaction had been born.
It turns out, however, that the fundamental particles were not alone: in the early twentieth century the physicist Paul Dirac showed that each fundamental particle comes with a twin called an antiparticle, which has the same mass but opposite electric charge. When two twins meet, they annihilate each other and their mass is transformed into energy. The concept of antiparticles originally dropped out of a mathematical equation which had one more solution than was expected, but since their theoretical conception antiparticles have actually been observed in cosmic rays and produced in laboratories.
Now the problem is this: whenever scientists have managed to produce antiparticles, they couldn't help but produce the corresponding particles in the process. The same must have happened during the Big Bang, so how come things didn't just annihilate each other and vanish in a puff of energy? Why do we see only matter and no antimatter?
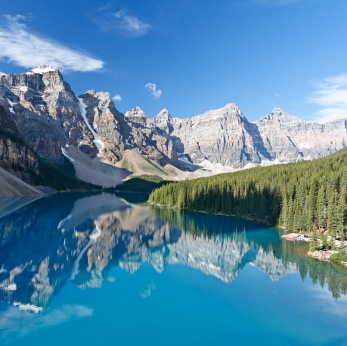
We're all familiar with mirror symmetry.
At the heart of this conundrum lies the notion of symmetry. Physicists traditionally believed that the laws of nature should be perfectly symmetrical: if you were to step into a mirror world unawares, you would never notice the difference, as the laws of physics should behave in just the same way there as they do in the normal world. In terms of particle physics, this means that whenever a particle or process exhibits "handedness", then the laws of nature should treat the left-handed version the same as the right-handed one. This is the concept of mirror symmetry, but there is a similar one, called charge symmetry, regarding matter and antimatter. It states that particles should behave exactly like their anti-twins.
But if charge symmetry prevails, how come we see no antimatter today? Could our antimatter-free world be the result of a violation of symmetry which caused young nature to "favour" particles over antiparticles, leading to the latters' eventual demise? This idea was first postulated in 1967 by Nobel Laureate Andrei Sakharov, who set out three pre-requisites for a world like ours to come into existence: one of them was that the laws of nature should indeed distinguish matter from antimatter.
The first proof that symmetry can in fact be broken had already been furnished in 1956, when the physicists Tsung Dao Lee and Chen Ning Yang proposed experiments that revealed a violation of mirror symmetry (and received the 1957 Nobel Prize in Physics as a result). Physicists at the time, however, clung on to the symmetry dream. They conjectured that while mirror symmetry and charge symmetry could be broken separately, the combination of the two would remain intact: if you were transported into an mirror world made of antimatter rather than matter, everything would behave just as you would expect. But in 1964 this last bastion of symmetry was shattered too: James Cronin and Val Fitch detected a violation of charge-mirror symmetry in the radioactive decay of strange particles called kaons (and in turn received the 1980 Nobel Prize in Physics for their work).
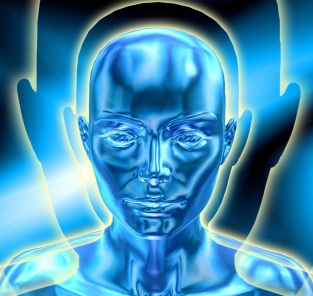
Charge-mirror symmetry: your anti-self in a mirror world should be just like you.
With the violation of charge-mirror symmetry now established by observation, what was still missing was the theoretical underpinning for this phenomenon — existing theories couldn't account for it. And this is where Kobayashi and Maskawa come in. They realised that the symmetry violation could be explained if one assumes the existence of three new types of fundamental particle. Once these are incorporated in the theory, the underlying mathematics obligingly works out to predict symmetry breaking. The hypothetical particles arising from their theory, known as charm quarks, bottom quarks and top quarks, were later observed in experiments. Kobayashi and Maskawa also predicted symmetry violation in other particles called B-mesons, and this was verified by experiment in 2001.
Why mass?
The second half of the 2008 Nobel Prize goes to Yoichiro Nambu for his work concerning another one of the big questions of particle physics: why do things have mass? Theory suggests that particles should have no mass at all, so how did they acquire it? What's more, why are their masses so different, with the heaviest, the top quark, more than 300,000 times heavier than the electron?
Perhaps surprisingly, Nambu's work towards an answer also involves broken symmetries, but this time they arise in connection with the vacuum. Technically, a vacuum is defined as a state with the lowest possible energy, not empty space as it is commonly thought of. As a very rough analogy, imagine yourself at your lowest possible energy state: slumped on the couch in front of the TV. You'll be relaxed, but chances are that you won't look symmetrical. To do that, you have to invest some energy in arranging your limbs and keeping them in place. Once you sit bolt upright with your muscles all tense, a small nudge would knock you out of position.
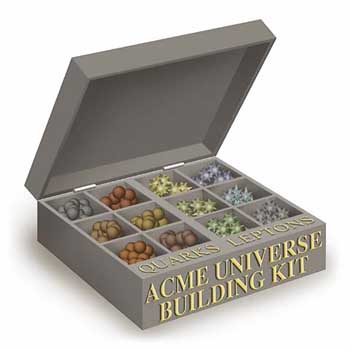
All matter is made up of a number of fundamental particles. But how did they acquire mass?
Similarly, the vacuum is a least energy state, but not a symmetrical state. Some sort of collapse of symmetry has occurred to get it into a low energy state, and because of its instantaneous nature, this collapse is known as spontaneous symmetry violation. Based on his previous work in superconductivity, Nambu created the mathematical tools to describe spontaneous symmetry violation in particle physics. He recognised the potential of studying broken symmetry in the vacuum, and his ideas form the basis for a whole body of work in the area. Among other things, they are frequently used to work out the effects of the strong nuclear force.
But what does all this have to do with mass? In 1964 the physicist Peter Higgs came up with a model for how particles acquired mass. He postulated that at the Big Bang an invisible force field, now known as the Higgs field existed, and it existed in a perfectly symmetrical state. But the Higgs field was unstable, and as the Universe cooled down, it slumped into an asymmetrical lowest energy state. As the zero-mass particles moved through the field, they interacted with it and experienced a drag, which we observe as mass. The mathematical tools created by Nambu paved the way for this theory of mass acquisition.
The three prize winners' work contributed significantly to our understanding of the Universe, but physics is by no means finished. The symmetry violations established by Kobayashi and Maskawa are far too small to account for the large-scale triumph of matter over antimatter, so more work needs to be done in this area. As for the Higgs field, the theory is still hypothetical. It is predicted that the field should come with its own messenger particle called the Higgs boson, which scientists will be looking for at the Large Hadron Collider at CERN. And while they're busy doing that, the rest of us can sit back and contemplate the curious fact that if it wasn't for a flaw in nature's symmetry, we wouldn't be here.
Further reading
There is a nice description of the prize winners' work on the Nobel Prize website.
There are also some relevant articles on Plus: