
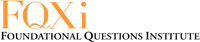
This article first appeared on the FQXi community website. FQXi are our partners in our Information about information project, within which we explored the concept of entropy. Click here to find out more about entropy.
The curious case of Benjamin Button tells the story of a man, played by Brad Pitt, who is born as an eighty-year old and grows younger as the years pass. The tale is obviously a fantasy — in reality, Brad Pitt is ageing. Angelina Jolie is ageing. You and I are ageing. As macroscopic objects, it's inevitable, and no amount of surgery can turn back the clock. But just why time only marches in one direction has long been a mystery.
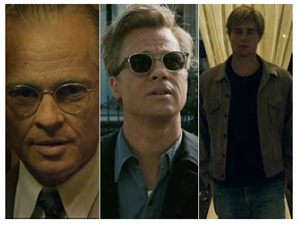
Brad's backwards body clock. (Image: Paramount/Warner Bros.)
Physicist Jonathan Dowling believes that quantum mechanics may hold the answer. At first sight, it may seem obvious to think that the ageing process is written into the movement of molecules in our skin. But on the microscopic scale, this movement is time reversible. If you look at the equations that govern how molecules interact with each other, they work just as well backwards as forwards. In other words, on the microscale, it's just as likely that shed skin particles should re-join our bodies as time passes, gradually making us look younger. Time's arrow could run backwards or forwards.
That doesn't happen. If you ask most physicists why not, they will refer to a law of physics that demands that the amount of entropy, or disorder, in the Universe always increases. When particles are shed from our skin, they go from being in an ordered state on our face to a disordered state as they join the rest of the dust in the room around us. While it is physically possible that these floating skin particles could each separate from the dust around us and re-join our face, it is far more likely that they will wander off in random directions. The arrow of time, in this conventional picture, comes from the understanding that while such processes could run backwards, it's vastly more likely that they won't.
But that explanation doesn't satisfy everyone. Dowling, of Louisiana State University in Baton Rouge, is searching for a more fundamental origin for this entropic arrow of time based on microscopic processes. "I'm not happy with the entropy explanation for reasons of simplicity," says Dowling. "I like to explain everything at the quantum level."
Early Love
Dowling's love of physics started early. "When I was in third grade, aged eight or nine, I went to a Catholic school in New York. One of the sisters asked the class what we wanted to be when we grew up. I stuck my hand up and said I wanted to be a physicist like Albert Einstein, and everyone laughed. So I thought, 'I'll show them!'"
And he did. Today, Dowling holds seven US patents and has over 150 scientific publications to his name. But he hasn't always pondered fundamentals like the origin of time's arrow. His first postdoctoral positions were in quantum optics at the Max Planck Institute, in Germany, and for the US Army where he worked on light-matter interactions in optical microcavities and the new field of photonic crystals. He shied away from foundational work when he finished his doctorate. "Someone on my PhD committee said I'd never get a job working on the foundations of quantum mechanics," says Dowling, "but that professor was wrong!"
Five years later, the tide turned as foundational quantum mechanics became extremely practical. Suddenly, Dowling's work had applications for sending secret messages using quantum cryptography and for making quantum computers. From this point on, he carved out a successful career in quantum technology, exploiting quantum entanglement for applications in quantum metrology, quantum sensing, and quantum imaging, amongst others.
Quantum Time
Seth Lloyd, an expert on quantum information theory at MIT, is a long-time fan of Dowling's. "He has a very loud voice, a great sense of humor," he says. "Dowling's done lots of important work on funky quantum effects, for instance for using quantum entanglement to do quantum lithography — the laying down of photons on a surface with high precision." Given Dowling's background, it's no surprise that he should look to fundamental quantum processes for an answer now that he is turning to the more esoteric problem of the origin of time's arrow. According to quantum mechanics, the motion of subatomic particles is independent of time's arrow until we sneak a peek at them by measuring them. "There's something odd going on when a measurement is made," says Dowling.
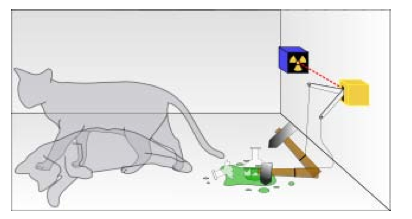
No going back. Prior to observation, Schrödinger's cat is both alive and dead. Opening the box seals its fate.
Consider Schrödinger's famous scenario in which an unfortunate cat is locked in a box with a vial of poison rigged to a hammer (see graphic, above). There is also a very small amount of a radioactive substance in the box and if one of its atoms decays, a relay mechanism will trip the hammer, break the vial, release the poison, and kill the cat. The quantum twist is that before we open the box and observe the state of the radioactive atom, the atom exists in a superposition of states: It has both decayed and not decayed. That means that the poison both has and has not been released. And so, before you look inside the box, the standard interpretation is to say that the cat is simultaneously dead and alive. Something strange then happens when you look into the box: the cat collapses into either life or death.
Resuscitating cats?
The key point for Dowling is that once the cat's fate is chosen, you cannot go backwards and resuscitate the dead cat: The arrow of time is set. This is a fundamental irreversible process in nature. With a grant of over $100,000 from the Foundational Questions Institute, Dowling will investigate whether this quantum measurement process could give rise to the arrow of time that we experience in our everyday lives.
To do so, Dowling must first get to grips with what exactly is happening when an observation is made and a quantum process is measured. He believes that irreversibility is intrinsic at the microscale, without the need for an observer. "People don't like this collapse business because it implies that human consciousness, in observing the cat, causes it to choose life or death," says Dowling. "I want to get around the collapse process using ideas from quantum information theory."
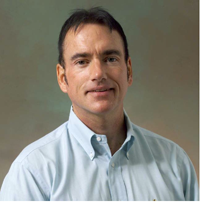
Jonathan Dowling. Louisiana State University.
In the process, Dowling will also butt heads with one of the biggest problems facing physicists: how to unite quantum mechanics with theories of gravity. Uniting these two theories has been the holy grail of physics for decades.
In theories of gravity, the arrow of time is set by entropy. According to cosmologists, the Universe started in a state of low entropy, and has been becoming more and more disordered overall, from the big bang onwards. Dowling hopes that he will be able to tease out how this large-scale cosmological phenomenon arises from fundamental quantum processes, as part of a larger programme to understand quantum gravity.
It's an audacious ambition. In the 1950s, physicists thought scaling up quantum theory to make it relativistic would be fairly straightforward for gravity. After all, it had only taken twenty years to develop a quantum theory of electric and magnetic fields. But sixty years on, it has proved a lot tougher to get a sensible quantum theory of gravity. Quantum optics expert Gerard Milburn of the University of Queensland, Australia, warns that this is a very speculative field of research, "largely due to the total lack of experimental evidence that we need such a theory."
So why doesn't Dowling take one of the more familiar approaches that physicists are currently using in an attempt quantise gravity, such as string theory or loop quantum gravity? Dowling's chief criticism is that these approaches are hung up on the physical embodiment of the theory, either loops or strings. Instead, with his background in quantum computing, Dowling plans to analyse the problem in terms of how information is encoded within quantum mechanical systems, just as conventional computer scientists analyse how information can be stored in bits.
"By using information theory we have a more general approach that is independent of the shape of the theory," says Dowling. "It frees you, allowing the information to take on a life of its own. I hope it's the Rosetta stone that takes us above the fray."
Lloyd likes this line of attack. "The idea of using quantum mechanics and information theory to approach questions of quantum gravity is an excellent one," he says. "Unlike more conventional approaches such as the loop theory of gravity and string theory, this goes to the heart of the paradoxes that have prevented scientists from constructing a successful theory of quantum gravity up to now."
Dowling faces the challenge of making quantum information theory consistent with Einstein's general theory of relativity. In non-relativistic quantum information theory, time is treated as a Newtonian concept, that is, there is one absolute time variable ticking along in the background that all observers share. However, in general relativity, time can run differently for different observers.
Entropy and Angelina
In his model of reality, Dowling eliminates the traditional concept of time ticking along at a uniform rate. Instead, he orders events in a causal way, replacing time with an ordering parameter or sequence. "Our hope is that by putting in ordering at the microscopic scale we will see entropy at the macroscopic scale," says Dowling. If he and his colleagues succeed, they will see an asymmetry in the way that events are ordered at the microscopic scale — the effects of increasing entropy on Brad and Angelina's molecules. Lloyd is optimistic: "Techniques of quantum information processing provide a potential solution to the problem of time, which has bedevilled quantum gravity from its inception in the 1920s," he says.
Dowling doesn't expect it to be easy, but bringing together different areas of research is always illuminating. "I'm very hopeful we'll learn new ways of looking at things," he says. "A lot of research is like scrabbling around in the dark looking for a few light bulbs. But we're having fun!"