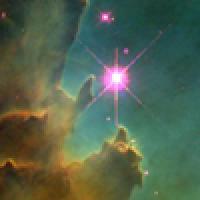
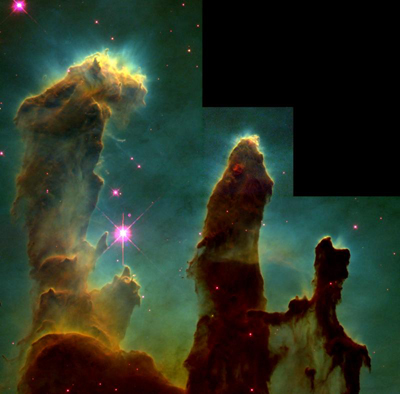
Gas pillars in the Eagle Nebula: One of Hubble's most famous images. Image courtesy NASA.
On May 19, 2009, the Space Shuttle Atlantis released the Hubble Space Telescope (HST) back into orbit after a hugely successful servicing mission, during which two new instruments have been installed on board the telescope, and two existing ones have been repaired. This marked the beginning of the next phase in the life of this incredible observatory. During its 19 years of operation, HST has produced 39 terabytes of data in the HST archive. At the time of this writing, 7,917 refereed scientific articles have been written based on HST data. With such a record, it is clearly impossible to even just list the HST's scientific accomplishments in a short article. In what follows, I have attempted to briefly describe (in no particular order) what I regard as the top five scientific discoveries. I should also note that rarely do astronomical discoveries belong to just one telescope. Usually it is many observatories, from the ground and from space, working in concert to produce a complete and multihued view of phenomena. Nevertheless, in the topics that I have selected, there is no doubt that HST played a crucial role.
The Hubble Constant
The astronomer Edwin Hubble, after whom the Hubble Space Telescope is named, determined in the 1920s that our Universe is expanding. The fabric of space between any two distant galaxies is stretching, just like the rubber of an inflating balloon. The rate at which galaxies are currently moving apart from one another is known as the Hubble constant, or H0. For a constant rate of expansion, the inverse of H0 gives the time at which the expansion started. But even in the presence of deceleration and acceleration H0 is the dominant factor in determining the age of the Universe.
Before HST, the value of H0 was known only to within a factor of two. The main reason for this large uncertainty was the fact that the determination of distances in astronomy is notoriously difficult. To overcome this obstacle, astronomers have constructed a distance ladder in which they use a series of standard candles — objects whose brightnesses are relatively well known — to infer distances to objects that are increasingly farther away.
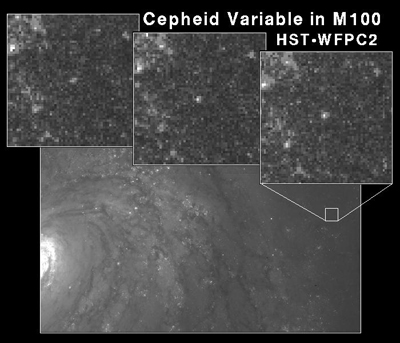
Figure 1: Cepheid variable star in galaxy M100. Image courtesy NASA.
Key standard candles are certain pulsating stars — stars that show periodic variations in their brightness — known as Cepheid variables. These are luminous stars, about a thousand times more luminous than the Sun, whose intrinsic brightness is tightly correlated to their pulsation period. By measuring the period of the variation, astronomers deduce the intrinsic brightness, and by comparing that to the apparent brightness, they can determine the distance (the apparent brightness decreases like the inverse square of the distance). The superb resolution of HST has allowed astronomers to isolate the light from numerous Cepheids in dozens of galaxies (figure 1), and the uncertainty in the value of the Hubble constant has initially been reduced to about 10%. Most recently, detailed HST observations of Cepheids in the galaxy NGC 4258 — whose distance is very accurately known through radio observations — coupled with observations of well-calibrated supernova explosions in more distant galaxies, have reduced the error in the value of H0 to less than 5%.
Dark Energy
In 1998, two teams of astronomers working independently discovered that the expansion of our Universe is in fact speeding up, propelled by the repulsive force of a mysterious dark energy. This came as a shock, because the prevailing assumption was that cosmic expansion should be slowing down due to the mutual gravitational attraction of all matter within the Universe. The precise nature of the dark energy that powers the acceleration is arguably the biggest puzzle that physics is facing today. Since the discovery, observations of the cosmic microwave background radiation have shown that dark energy makes up more than 70% of the total energy density of the Universe. The Hubble observations relied on a particular type of supernova explosion (known as Type Ia supernovae) to trace the expansion history of the Universe (figure 2), and thereby to place constraints on the properties of dark energy. There are two factors that make type Ia supernovae particularly useful in this regard. First, they are extremely bright, and therefore can be observed half way across cosmic time. Second, they are good standard candles — their luminosities are nearly constant — and therefore their distances can be determined quite accurately. Hubble's sharp vision has allowed astronomers to pinpoint distant supernovae in galaxies that are as far as nine billion light-years away, and to determine that dark energy was already present (though not dominant) even at that early stage, when gravity still had the upper hand and the expansion of the cosmos was decelerating.
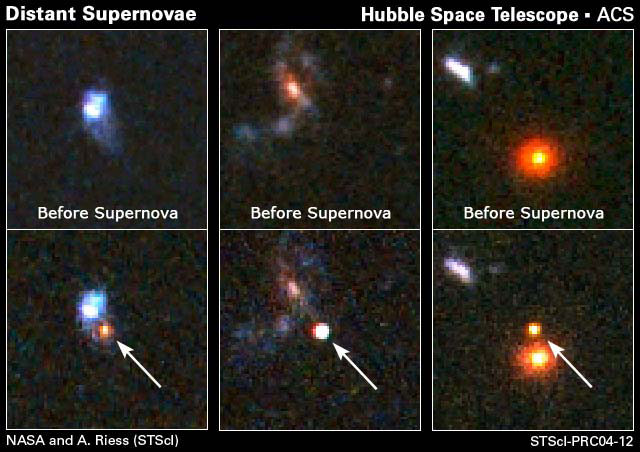
Figure 2: Hubble spots distant supernovae in search of properties of dark energy. Image courtesy NASA.
All the observations to date are consistent with dark energy being the energy of empty space. Quantum mechanics, the theory that most accurately describes the sub-atomic world, says that the physical vacuum, far from being empty, is teeming with virtual particles that appear and disappear in split seconds. The energy density associated with the vacuum is constant, and characterised by an equation of state parameter w (the ratio of pressure to density), which, according to the theory, satisfies w = -1. Most of the current observational efforts are directed at determining whether w is indeed constant across cosmic time, and whether its value is equal to –1. However, even if the characteristics of dark energy will be found to be fully consistent with it being the energy of the vacuum, many open questions will remain. In particular, naive attempts to theoretically calculate the expected value of the vacuum energy density produce results that are more than 50 orders of magnitude higher than the observed value (see Plus article Lambda marks the spot for more information). Consequently, a true understanding of dark energy will require a combination of observational efforts coupled with significant theoretical developments.
Galaxy formation and evolution
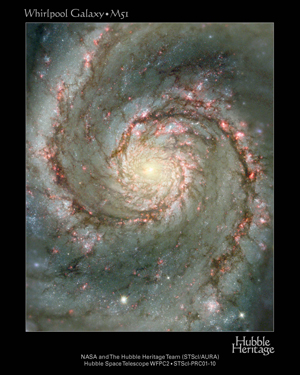
Figure 3: The heart of the Whirlpool Galaxy. Image courtesy NASA.
Everyone is familiar with the shapes of the galaxies that we see in the relatively local Universe. Galaxies such as the Milky Way and the Andromeda galaxy are disc galaxies: they are flattened like pancakes, and are characterised by a prominent spiral structure traced by young stars (figure 3). Other galaxies are elliptical: they are shaped like oval concentrations of relatively old stars. One of the key goals of astronomy is to understand how galaxies form and how they evolve. Astronomers using HST produced the deepest images of the Universe in optical light. These observations were dubbed the Hubble Deep Fields and the Hubble Ultra Deep Field (figure 4). The observations revealed that galaxies in the distant past were smaller in physical size, and that their shapes were much more irregular. These two properties are consistent with a scenario of hierarchical structure formation, in which smaller building blocks of galaxies collided and coalesced frequently in the early, dense Universe, to form the larger galaxies we observe today. Using HST's exquisite spatial resolution and photometric stability, astronomers were also able to observe in detail the stellar populations in the old halos of nearby galaxies, which allowed them to reconstruct how mass was assembled in these galaxies.
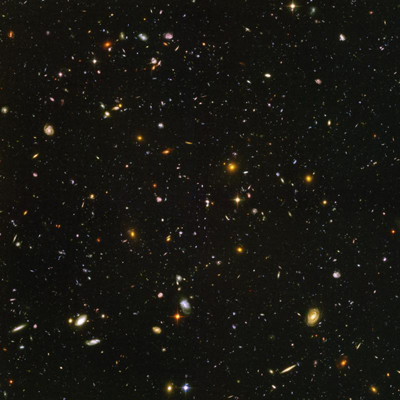
Figure 4: The Hubble Ultra Deep Field image reveals galaxies galore. Image courtesy NASA.
Finally, the deep-field observations yielded the history of the global rate of star formation in the cosmos. Even before the Hubble observations, astronomers knew that our Universe as a whole is past its peak in terms of the rate of birth of new stars. The peak occurred about 7 to 8 billion years ago. The Hubble Ultra Deep Field showed that when the Universe was less than one billion years old (the Universe today is 13.7 billion years old) the rate of cosmic star formation was lower than the peak value by about a factor of three, but already higher than the rate today, which is about a factor of ten lower than at the peak. In other words, once the Universe started forming stars, it did so furiously.
Supermassive black holes
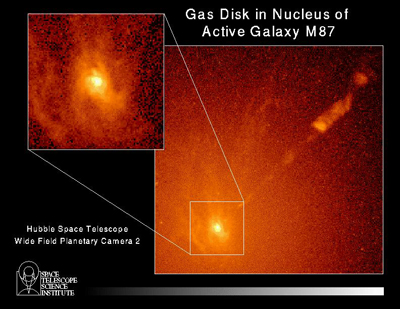
Figure 5: Hubble confirms the existence of a massive black hole at the heart of an active galaxy. Image courtesy NASA.
Astrophysicists have long suspected that active galactic nuclei — the extremely bright cores of galaxies that show violent activity — and quasi-stellar objects (QSOs) create their extraordinarily high luminosities by accreting mass at a high rate onto a black hole that lies at their centre. The power emitted simply reflects the rate of release of gravitational potential energy. However, before HST it was virtually impossible to detect the host galaxies of QSOs, and it was extremely difficult to confirm the presence of a black hole, except in a couple of relatively nearby galaxies, and in our own Milky Way Galaxy. HST changed all that. First, it has unambiguously detected the host galaxies in a few relatively nearby QSOs. Second, by following the motions of individual stars (or of gas discs) around the centres of tens of galaxies, it has shown that essentially all the galaxies that have a central bulge of stars harbor a supermassive black hole at their centres (figure 5). The masses of these black holes range from a few millions to a few billions of solar masses. But in addition to the mere discovery of the black holes, HST provided two other significant pieces of information. First, high-resolution images of the hosts of QSOs revealed that many of them were interacting galaxies and the others were bright elliptical galaxies. This suggests that certain environments (such as the one resulting from an interaction) may be needed to funnel gas into the central regions to fuel the black holes.
Second and more important, the masses of the black holes were found to be tightly correlated with the masses of the smooth, spherical bulges of stars surrounding the galactic centres. This indicates that the black holes and their host galaxies do not evolve independently, but rather that their evolutions are intimately connected — massive black holes are apparently a generic feature of galaxy formation and evolution.
Extrasolar planets
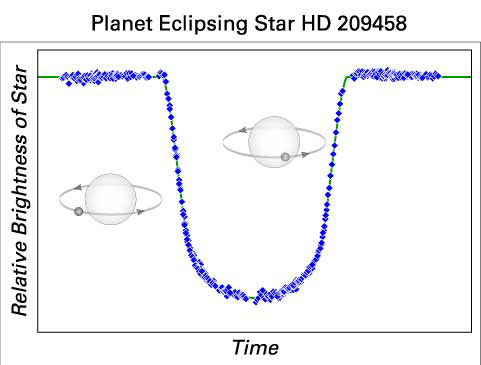
Figure 6: A planet's telltale signature. Image courtesy NASA.
Until 1992, we did not know of a single planet outside our solar system. In 1992, the first so-called extrasolar planets were detected, but they did not orbit an ordinary star such as the Sun. Rather, they were found around a pulsar — an extremely compact object with a mass of about 1.4 solar masses, but a radius of only about 10km. The first planet around a Sun-like star was discovered in 1995. Since then, astronomers have discovered about 350 extrasolar planets. Most of these planets were discovered by ground-based telescopes. Still, HST has contributed a few unique observations to this field. First, Hubble focused on transiting planets — planets whose orbital planes are aligned with our line of sight, so that the planets periodically eclipse their host stars. When the planet passes in front of the star, it blocks some of the star's light. From the amount of dimming (typically 1-2%) the radius of the planet can be deduced (figure 6). But this is not all. Some of the starlight passes through the planet's atmosphere, where part of it is absorbed by various atoms. By concentrating on particular spectral lines, the presence and abundance of certain atoms and molecules can be determined (see Plus article Hunting for life in alien worlds for more on this technique). In this way, HST observations showed that the atmosphere of the planet around the star HD 209458 contains sodium, carbon, oxygen, and hydrogen. In another case, even water and methane were detected. These were the first determinations of the composition of atmospheres of extrasolar planets.
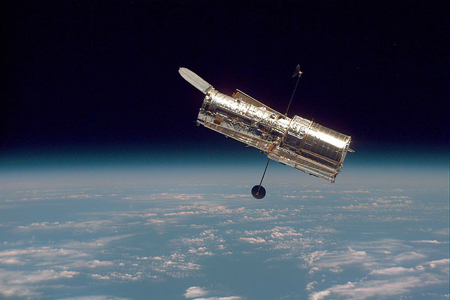
The Hubble Space Telescope against the Earth's horizon. Image courtesy NASA.
Most of the extrasolar planets were found around stars in the solar neighborhood. This still left open the question of whether the local fraction of stars hosting planets is typical of the Galaxy at large. To answer this question, HST observed about 180,000 stars in the crowded central bulge of our Galaxy, half-way across the Milky Way. These observations led to the discovery of 16 planet candidates, a tally consistent with the frequency of planets in the solar neighborhood, and they showed that the Galaxy is indeed teeming with billions of planets. Five of the planet candidates were found to whirl around their stars in less than one Earth day, and they were dubbed Ultra-Short-Period Planets.
Finally, HST produced the first visible-light snapshot of a planet orbiting another star. The planet, known as Fomalhaut b since it circles the bright southern star Fomalhaut, was resolved inside a large debris disc, somewhat similar to the Kuiper Belt in our own solar system. The planet's distance from its host star is about ten times the distance of the planet Saturn from the Sun.
The Future
Following Servicing Mission 4, Hubble has the largest complement of functioning instruments it has ever had. Assuming that the Wide Field Camera 3 (WFC3), the Cosmic Origins Spectrograph (COS), the Advanced Camera for Surveys (ACS), and the Space Telescope Imaging Spectrograph (STIS) will work as expected, many Hubble discoveries are still to come. In particular, WFC3, with its infrared capabilities, will take us even closer to the very first galaxies, and COS will reveal to us the structure and composition of the cosmic web — the filamentary intergalactic gas. You can be almost certain that five years from now, the list of the top five Hubble discoveries will have to be revisited.
About the author
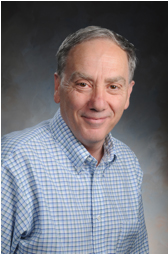
Mario Livio is a theoretical astrophysicist at the Space Telescope Science Institute, which conducts the scientific program of the Hubble Space Telescope. He has published more than four hundred scientific articles in a wide range of topics in astrophysics. Dr Livio's recent book, Is god a mathematician? was reviewed in Issue 49 (December 2008) of Plus.
Comments
really helped me understand
really helped me understand astrophysics for school! :) thank you
school
really helped me in my assignment
sicence
helps with my project about the solar system
Permalink helped me with my assignment
The information in this article was very informative.