
What could be more dependable than the daily cycles of the Sun, Moon and the other planets in our solar system? Not much, you might think, but strictly speaking you'd be wrong. Since the 1980s scientists have suspected that the motion of the solar system is chaotic. Now they have found evidence that this is really the case — not in the sky, but in a North American rock formation.
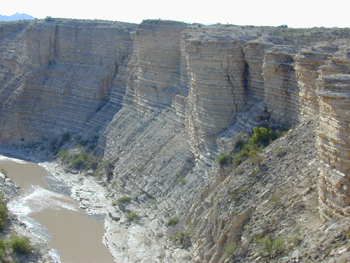
This layer cake of sedimentary rock near Big Bend, Texas, holds a signature of chaos. Image: Bradley Sageman, Northwestern University.
The idea of a chaotic solar system sounds quite shocking at first. Naively, you might think that something as seemingly eternal as the solar system would be reasonably robust. If one of the planets received a tiny little push, for example, you might expect a small variation in its orbit, but nothing more. In a chaotic system, however, even the tiniest variations can snowball over time, leading to drastic changes. A pushed planet, even if knocked only ever so slightly, could end up leaving its orbit completely and eventually exit the system or crash into another planet. (You can see an animation of such a scenario here.)
This phenomenon is know as the butterfly effect, or, more technically, sensitive dependence on initial conditions. We see it every day in meteorology: the butterfly effect is the reason why weather forecasts are only accurate for the very immediate future and then become unreliable very quickly. If the forecasters get today's conditions just a tiny little bit wrong — which they are bound to because they can't work with infinite accuracy — then they will miss out on tiny variations that may turn into extreme weather in places they thought would be warm and sunny. For the solar system the time period over which small variations balloon is much longer, tens of millions of years or more. So save some unexpected external influence, we won't see the planets veer off their orbits in our life times and far beyond.
But even if it won't affect us, the question of whether the solar system is stable or not has been fascinating scientists at least since the time of Newton. Using Newton's laws you can calculate the trajectory of a single planet orbiting the Sun: it has the shape of an ellipse, which is represented by a neat mathematical formula. When you add in a second planet (or star, or asteroid, or any kind of body), however, things become a lot more complicated. Not only do you have you take account of the gravitational pull of the Sun on the bodies, but also of the bodies on each other. At first sight this doesn't seem to matter in our own solar system. The masses of the planets are so small compared to that of the Sun, that their mutual pull is almost negligible — which is why the planets orbit the Sun in nice, apparently elliptical orbits. But could it be that the tiny variations resulting from the planetary interactions could build up over time and eventually destroy the whole system?
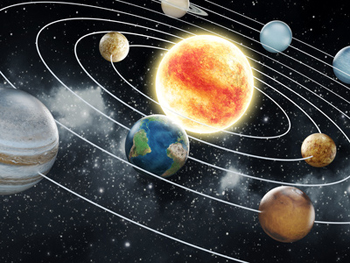
It might not look chaotic, but evidence suggests that it is.
It's a question that loomed large in the 18th century, especially because observations showed that Jupiter was moving closer to the Sun, while Saturn was moving away from it. At the end of the 19th century King Oscar II of Sweden and Norway launched an international maths competition to settle the question once and for all. The task was to describe the behaviour of any planetary system using Newton's laws of motion and gravitation: you're given a bunch of (possibly imaginary) planets with particular locations and masses, and your task is to use Newton's equations to calculate their trajectories. A young French mathematician by the name of Henri Poincaré took up the challenge, won the prize, and promptly discovered a major flaw in his argument. This put him in an embarrassing position since his manuscript was to be published for the King's birthday within a few weeks' time. Honourably, Poincaré admitted his mistake and a new manuscript was published a year later.
In his work Poincaré restricted himself to systems containing only three planets. Perhaps shockingly, he proved that there's no general formula (like, for example, that describing an ellipse) that hands you the solution on a plate for all possible configurations of planets. The planets' trajectories don't have to be nice and regular as that of the Earth, but can be complex, wild, and apparently random. In fact, Poincaré discovered that a celestial system can depend sensitively on initial conditions: it can be chaotic.
Despite this fact, the hope that our solar system might be nice and regular persisted until well into the twentieth century. In 1961 the mathematician Vladimir Arnold proved that a large proportion of possible solar systems will still behave in a predictable manner (quasiperiodically in technical terms) if the masses, eccentricities and inclinations of the planets are sufficiently small. The real solar system doesn't satisfy Arnold's conditions, but people thought the result might also apply beyond its technical limits.
The hope was dashed with the advent of modern computers, which were powerful enough to provide better predictions based on realistic models of the solar system. In 1988, it became clear that the motion of Pluto, then considered a planet but now demoted to a dwarf planet, was chaotic. This didn't matter too much for the rest of the system because Pluto's mass is so small. But a year later, a model, devised by Jacques Laskar suggested that the same holds true for the entire solar system. In Laskar's model an error of 15 metres in the initial location of the Earth grows into an error of 150 metres after ten million years, and then into a huge error of 150 million kilometres after 100 million years.
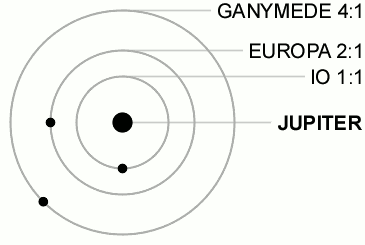
An illustration of resonance between three of the moons of Jupiter; Io, Ganymede, and Europa.
What was missing until now was some hard evidence for the chaos in the solar system. A signature of chaos we can measure, here on Earth or in the sky. Such a signature comes from resonances between planets: if the orbits of two planets around the Sun are in tune with each other, they periodically come close enough to exert a notable gravitational pull on each other. If the solar system is indeed chaotic, then, so models predict, the chaos should have led to a drastic disruption in the resonance between Mars and Earth somewhere between 50 and 100 million years ago. And because resonances can have an effect on a planet's location and tilt on its axis, they can effect the amount of sunlight a planet gets, and therefore its climate. A resonance transition can leave traces in the Earth's rocks, whose make-up depends on climate.
That's exactly the smoking gun that has been found in the Niobrara Formation in North America. "The Niobrara Formation exhibits pronounced rhythmic rock layering due to changes in the relative abundance of clay and calcium carbonate," says Stephen Meyers, one of the scientists involved in the new research. "The source of the clay (laid down as shale) is from weathering of the land surface and the influx of clay to the seaway via rivers. The source of the calcium carbonate (limestone) is the shells of organisms, mostly microscopic, that lived in the water column. [...] Climate change influences the relative delivery of clay versus calcium carbonate, recording the astronomical signal in the process."
This signature of the Earth-Mars resonance transition is around 87 million years old. It indicates that there really is chaos in the solar system, and also helps researchers pin-point which of the various chaotic models is the right one. "Other studies have suggested the presence of chaos based on geologic data," says Meyers. "But this is the first unambiguous evidence, made possible by the availability of high-quality, radioisotopic dates and the strong astronomical signal preserved in the rocks." The research has been published in the journal Nature.
Comments
correction needed
The planets' trajectories don't have to be nice and regular as that of the Earth, but can WE complex, wild, and apparently random.
Needs to be changed to
The planets' trajectories don't have to be nice and regular as that of the Earth, but can BE complex, wild, and apparently random.
Thanks for spotting that typo
Thanks for spotting that typo!