
Phase transitions are best illustrated by a substance we come across almost every day: ice. Ice is so familiar to us that we just accept the sudden and dramatic metamorphosis of water as it cools below its freezing point. A liquid that we can dive into is spontaneously transformed into a hard rock-like material that would crack our skulls.
The miraculous transformation that turns liquid water into ice is known to physicists as a phase transition. Many other phase changes are known to physics where, at a well-defined temperature, the structure of a material changes completely. These include the condensation of a gas into liquid, the transformation of liquid helium into a superfluid and the transition of a normal conductor into a superconductor, which happens in a range of materials that lose all electrical resistance at low temperatures. There are many other examples too.
Model magnets
How do phase transitions occur? For a concrete example, imagine a magnetic material, such as iron, nickel and cobalt. These materials undergo a phase transition at a temperature known as the Curie temperature, after the French scientist Pierre Curie, husband of the famous Marie Curie. The Curie temperature of iron is 1043K, which corresponds to 770 degrees Celcius. Above this temperature a lump of iron will lose its magnetism, while below this temperature the iron will spontaneously magnetise.
As a simplified model of a magnet, imagine a collection of atoms arranged regularly in a plane, each atom having a small magnetic field that is constrained to point in one of two directions: it can either point upwards or downwards.
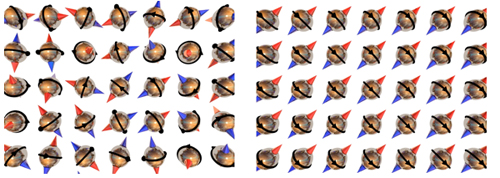
Figure 1: Schematic depiction of the atoms in a ferromagnet. Left: The symmetrical phase above the Curie temperature where all the magnetic fields of the atoms are randomly oriented. Right: The asymmetrical phase below the Curie temperature where the magnetic fields of all the atoms are aligned in the same direction.
According to the laws of thermodynamics, such a system quickly settles in an equilibrium: that's a state of lowest energy. The energy of two neighbouring atoms is lowered slightly if their magnetic fields point in the same direction, so there is a tendency for them to align. However, at high temperatures thermal vibrations vigorously jostle the atoms around with the effect that their magnetic fields are continually being flipped. So, although there might be a tendency for neighbouring atoms to align their magnetic fields, any such alignments are rapidly disrupted. In this state the material will not exhibit a magnetic field macroscopically, as the randomly oriented magnetic fields of all the atoms cancel each other out.
But when the temperature is lowered, thermal vibrations are no longer strong enough to disrupt all of the alignments and most of the magnetic fields will align in the same direction. The material will now have a macroscopic magnetic field. It has become a magnet. As the temperature is lowered further beyond the critical point, the size of the thermal vibrations will decrease to the point where they are so small that even the biggest will not flip atoms into the wrong direction. All the atoms will now be pointing in the same direction.
When water freezes to ice, something quite similar occurs: the water molecules that were previously moving around quite randomly suddenly settle into a rigid grid as the temperature becomes cold enough.
Interestingly, the phase transition in the magnet is connected to a loss of symmetry. Above the critical temperature the direction of the magnetic fields of the atoms in a magnet are arranged completely at random. The magnetic field of each atom is equally likely to be pointing in either direction, so the system does not distinguish between the two directions, they are completely equivalent. In this state the system is symmetrical with respect to the up and down directions. But below the critical temperature this symmetry has disappeared as the magnetic fields of most of the atoms are now pointing in the same direction.
Such a loss of symmetry occurs in many phase transitions, including water freezing to ice. This was realised in the 1930s the Russian theoretical physicist Lev Landau. The insight was the first step towards building a mathematical model of phase transitions. To this day mathematicians are hard of work using the power of mathematics to better understand those dramatic changes we observe in nature.
This article is an excerpt of our article Secret symmetry and the Higgs boson by Nicholas Mee.
This article forms part of our collaboration with the Isaac Newton Institute for Mathematical Sciences (INI) – you can find all the content from the collaboration here.
The INI is an international research centre and our neighbour here on the University of Cambridge's maths campus. It attracts leading mathematical scientists from all over the world, and is open to all. Visit www.newton.ac.uk to find out more.
