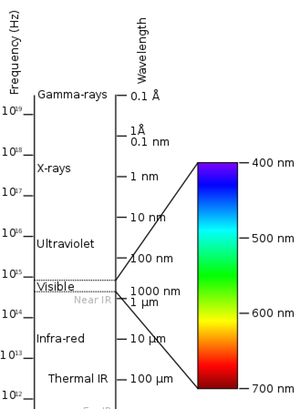
The spectrum of electromagnetic waves, including the visible light range. (Image Victor Blacus – CC BY-SA 3.0)
The 2018 Nobel prize in physics has been awarded to Arthur Ashkin "for the optical tweezers and their application to biological systems" and to Gérard Mourou and Donna Strickland "for their method of generating high-intensity, ultra-short optical pulses". These inventions have revolutionised laser physics and had wide ranging applications in science, technology, industry and medicine.
Light consists of electromagnetic waves. The wavelength $\lambda$ is related to the frequency $f$ of a light wave by the formula that works for all waves, $\lambda = c/f$, where $c$ is the speed of the light wave, around $3\times 10^8 ms^{-1}$. The wavelength and corresponding frequency of the wave determine the colour of the light we see in the visible spectrum.
Naturally occurring light consists of light waves of many different wavelengths scattering in every direction. The light waves in a laser are different. They are coherent: they have the same wavelength, they travel in the same direction, and they are in phase – the peaks and troughs of the waves line up perfectly.
Cloning light
The identical waves can be created thanks to the dual nature of light that was first proposed by Albert Einstein in the beginnings of quantum physics. Light can simultaneously act like a wave and act like a particle called a photon. (You can read more in Light's identity crisis.)

The Bohr model of the atom. Electrons are only permitted to be at certain distances from the centre. (Image: JabberWok – CC BY-SA 3.0)
Photons can be generated by the quantum behaviour inside atoms. Most of us have in our head a solar system model of an atom held together by electrical rather than gravitational forces. This picture was refined by Niels Bohr in 1913 when he proposed that electrons could not orbit the nucleus of an atom at any distance: only certain distances are allowed. The distances are related to the energy of the electron, with lower energies corresponding to the electron being nearer the nucleus. When an electron loses energy, it doesn't gradually inch inwards towards the nucleus, but performs a leap — a quantum jump — to the next allowed distance. And when an electron performs such a leap the energy it loses is released as a photon. (You can read more in Quantum uncertainty and Why quantum mechanics?.)
Due to the dual nature of light this photon can also be seen as a wave with a frequency and corresponding wavelength, and these are determined by the amount of energy lost by the electron that created the photon: $$ E=hf=\frac{hc}{\lambda} $$ where $h$ is Planck's constant.
Lasers start with a material made up of the same type of atoms that is primed by some external energy source so that enough electrons are in specific high energy orbits around the nuclei. Then, if the material is in a particular state, something called stimulated emission occurs. A photon generated by the drop of one electron between orbits moves through the material. As it passes through another atom with an electron in the specified high energy orbit, it causes that electron to drop and another photon is released, with the same energy (and hence frequency and wavelength) as the first photon. Now we have two photons moving through the material which not only have the same wavelength, they are also moving in the same direction and their waves are in phase.
This process repeats over and over as the growing number of cloned photons move through the material. Mirrors are placed on either side of the material to amplify this effect. And the stream of cloned photons is released as a beam of laser light through a small transparent patch in one of the mirrors.
Light trap
The coherence of these cloned photons means that it is possible to control the beam of light from a laser very precisely. For example the beam can be kept very tight, rather than dispersing in all directions like the beam of light from a torch. Lasers can travel huge distances – they have been used to accurately measure the distance between the Moon and the Earth. And as well as enabling scientific advances, lasers are now part of our everyday lives. For example, they are used to store and read the information stored as microscopic pits in the surface of a CD or DVD and are used in barcode scanners at the supermarket. This year's Nobel prize in physics recognises two developments that have revolutionised the use of lasers.
When the first lasers were built in the 1960s, Ashkin began to experiment with using them to move small particles. He was successful, but to his surprise he also discovered an unexpected behaviour – the particles were drawn to move along the centre of the laser beam where the light was most intense. This was explained by the pressure the light exerted on the particle varying with the intensity of the beam (which was greater at the centre, reducing to the weakest at the edge of the beam): the sum of all these forces pushes the particle to the centre.
When Ashkin added a strong lens to focus the beam, the particles were drawn to and trapped in the spot where the light was most intense. Over several years Ashkin was able to develop the technique until these optical tweezers could capture atoms. This tool has had many applications but Ashkin’s own application has also been recognised by the Nobel committee. He was able to use optical tweezers to capture living bacteria without harming them, creating a new way to study biological systems now widely used in medical and biological research.
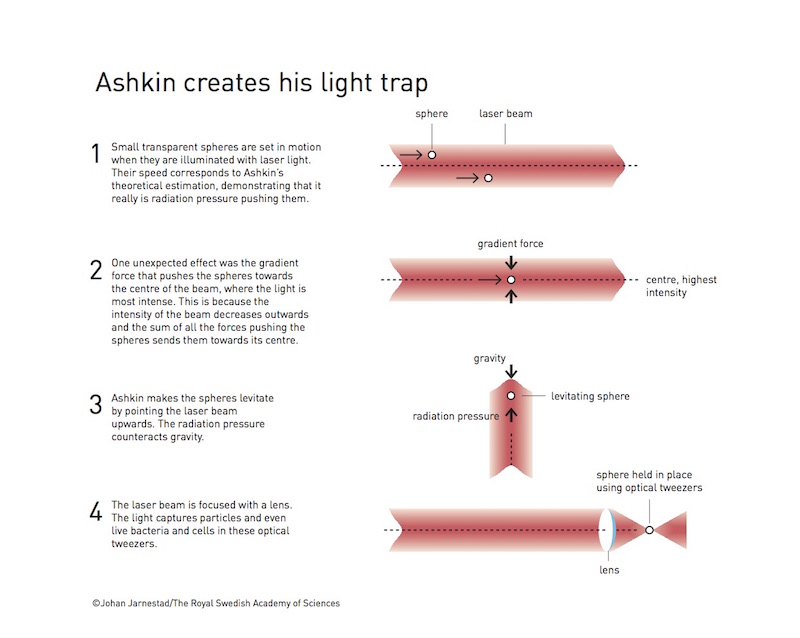
Boosting light
Strickland and Mourou's breakthrough appeared in Stickland's first scientific publication in 1985 when Mourou was her supervisor. Lasers can be used to emit short bursts of light and researchers were keen to find ways to find ways to increase the intensity of these pulses. After an initial period of success in increasing the power of laser pulses since lasers were first developed, progress plateaued. By the mid 1980s researchers had reached the limit of how much they could amplify a laser pulse without damaging the amplifiers they were using.
Strickland and Mourou came up with an ingenious technique that is now known as chirped pulse amplification (CPA). They first stretched out a short laser pulse, so that its peak power would be lower. They then passed this lower power, longer pulse through the amplifier. And finally, they squeezed the pulse back to its original length, dramatically increasing the power of the final pulse.
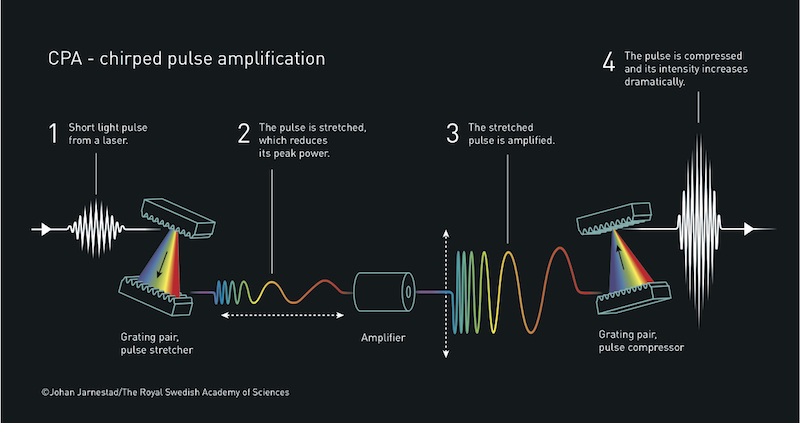
Strickland and Mourou's CPA technique is now used throughout science and industry to produce high-intensity lasers. It has had many applications and enabled new areas of physics, chemistry and medicine, include laser eye surgery, which is now routine and enhancing the vision of many people every day.
The work of Ashkin, Mourou and Strickland really does fulfil Alfred Nobel's wish that the prizes recognise work that has been of the "greatest benefit to mankind". The Nobel prize in physics this year is also very welcome as it is the first time it has been awarded to a woman for 55 years, and only the third to be awarded to a woman. When this was pointed out to Strickland during the press conference she responded: "Is that all, really? I thought there might have been more." Perhaps the Nobel prize will amplify her positive experience as a physicist and encourage the recognition of others. "We need to celebrate women physicists because we’re out there. Hopefully, in time, it will start to move forward at a faster rate."