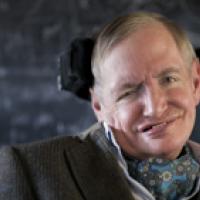
This article first appeared as a feature on the website of the Cambridge Mathematics Faculty.
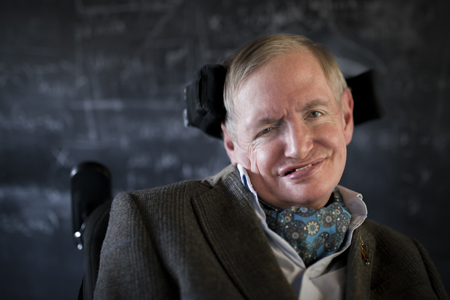
Stephen Hawking.
Ask a 12-year-old child to explain the Big Bang or a black hole and chances are you'll get a reasonably correct answer. Ask a physicist about the same concepts and he or she will tell you they are accepted components of our theory of the Universe. When Stephen Hawking started his career in the early 1960s neither of these two statements was true. The fact that they are now is to no small extent due to Hawking himself.
At the beginning of 1960s the idea that the Universe might have sprung from an extremely hot and dense beginning — a Big Bang – was hardly more than scientific speculation, much like the entire field of cosmology was barely considered a legitimate part of science. The then accepted theory of the history of the Universe, the steady state theory, held that the Universe had existed forever. Indeed, had Hawking been allowed to study under the PhD supervisor of his choice, the astronomer Fred Hoyle, he would have been drawn into defending this theory. As it happened, he helped to demolish it.
From the Big Bang…
The discovery of the cosmic microwave background (CMB) radiation in 1964 provided the first nail in the coffin of the steady state theory. The theory couldn't explain the existence of this faint glow that permeated the Universe, but Big Bang theory could: the CMB might be nothing less than left over radiation from the Big Bang.
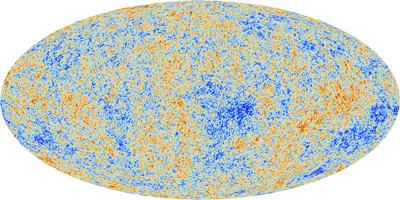
A map of the cosmic microwave background (CMB) formed from data taken by the Planck spacecraft. The varying colours represent tiny variations of the temperature of the CMB. These variations that give important clues about the nature of the Universe. Image: ESA and the Planck Collaboration.
Having begun his studies under Dennis Sciama instead of Hoyle, and influenced by the eminent Roger Penrose, Hawking began to investigate whether the general theory of relativity necessarily implied that the Universe started in an initial singularity. Another alternative to the steady state theory was that of a cyclical Universe, which eternally contracts to a hot and dense Big Bang-like state and then expands again. Working with George Ellis and Roger Penrose, Hawking eventually arrived at his famous singularity theorem: according to general relativity, an initial singularity was not only possible, it was inevitable. By the beginning of the 1970s Big Bang theory was fully accepted. Apart from its theoretical foundations, the Big Bang even owes its name to Hawking.
...to black holes
The Big Bang wasn't the only inevitable singularity, however. Hawking's work with Penrose showed that singularities would also occur inside black holes, regions of space within which the gravitational pull is so strong that nothing, not even light, can escape (or so it was thought). Black holes can arise, for example, when a massive star collapses under its own gravity.
Hawking's work on black holes, a natural step on from the Big Bang, established our current understanding of these gravitational monstrosities. Among other things, he discovered the notion of an event horizon of a black hole, the boundary of no return that surrounds the central singularity. He also proved his famous area theorem, which states that, no matter what happens to a black hole, the area of its event horizon can never become smaller. This hinted to a curious connection to a different part of physics, picked up by the young physicist Jacob Bekenstein: in thermodynamics there is a measure of the disorder of a physical system, called its entropy, which also never decreases. But since black holes were thought to be simple objects that didn't come with any degree of disorder — they were just black — this was thought a mere analogy without deeper meaning.
After a good run of work on black holes by Hawking and others, Hawking thought the field was all wrapped up. "I remember Stephen coming back [from a conference in 1973] and saying the subject is finished, we should look for another problem," says Gary Gibbons, Professor of Theoretical Physics at the Department of Applied Mathematics and Theoretical Physics and former student and collaborator of Hawking's. "So we started looking at a suggestion that he had made earlier: that there should be very small black holes formed in the early Universe. You should think of them as fossils of the Big Bang. If you could find them they would provide an important clue to the origin of the Universe."
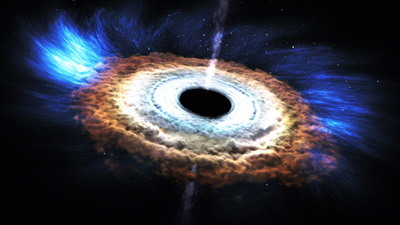
A star is torn. This artist's impression shows a star approaching too close to a massive black hole and being torn apart by tidal forces. Image courtesy NASA's Goddard Space Flight Center/CI Lab.
Little did Hawking know at the time that these fossils' failure to exist would lead to his most celebrated result in black hole theory. While working with Gibbons, Hawking realised that even neutral, non-rotating primordial black holes would lose mass and would have evaporated long before our time. This amounted to a revolutionary statement. It said that black holes aren't completely black, but can emit thermal radiation — that's how they evaporate. The idea showed that black holes were in fact thermodynamic objects and turned Bekenstein's analogy to thermodynamics into a reality (you can find out more here). Hawking went on to formulate a thermodynamic theory of black holes, the central equation of which is the famous Bekenstein-Hawking entropy formula. "I would like this simple formula to be on my tombstone," Hawking said in his 60th birthday address.
Hawking's results on black holes were theoretical in nature. It wasn't until 2015 that the spectacular detection of gravitational waves opened up the possibility to test some of Hawking's results, including the area theorem, experimentally. "The data is not yet good enough to do this," Hawking said at his 75th birthday address last year. "But in the near future it should be possible [...]. With many more gravitational wave detections expected [...] I am excited by the possibilities the new era of gravitational wave astronomy will bring."
One theoretical problem about black holes, which has been causing physicists sleepless nights for decades, still remains open. If an object falls into a black hole, is the information that comes with it lost, or can it be recovered from the radiation when the black hole evaporates? If it were lost then a fundamental law of physics, the second law of thermodynamics, would be violated. This information paradox hugely intrigued Hawking. During the last years of his life Hawking, Malcolm Perry and Andrew Strominger worked hard on a mechanism that explains how information may be recovered.
From black holes to the whole Universe
Hawking's work has consequences for all of cosmology and even the whole of physics. Just as the singularity theorems applied to the "local" phenomenon of black holes and the "global" phenomenon of the Big Bang, his black hole radiation result may also illuminate the history of the Universe in its entirety.
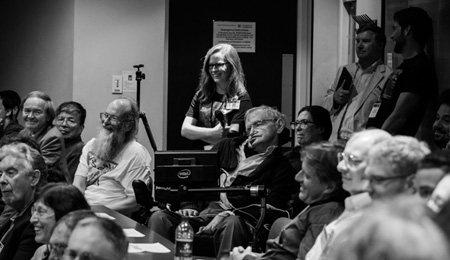
Stephen Hawking and colleagues attending a talk at Hawking's 75th birthday symposium. Image: Tobias Baldauf.
Remarkably, Hawking and Gibbons realised that the Universe as a whole could, in some sense, be regarded as a black hole turned inside-out. One of the major mysteries about the Universe at the time was how the large-scale structures we see today — planets, stars, galaxies — came into being. The Hawking-Gibbons idea of an inside-out black hole helped make precise a theory that resolves this mystery. The theory explains how quantum fluctuations that occurred at a time when the Universe was undergoing a period of rapid expansion could have seeded the large structures we see today. That period of expansion was called inflation and was first suggested by the physicist Alan Guth.
Fanciful as it may sound, the idea is more than just pure theory. The theory of inflationary fluctuations now has excellent observational evidence, particularly from the cosmic microwave sky as observed in increasing precision by the COBE, WMAP and Planck satellites. Physicists hope that current and future experiments looking for the signatures left by gravitational wave in the CMB will offer further insights about the nature of inflation and early epochs of the Universe, and even about the fundamental structure of the Universe.
The biggest challenge?
In terms of physics as a whole, Hawking's work is significant because it links two areas that up to now have refused to coexist in harmony. By their very definition black holes are linked to the force of gravity and therefore described by general relativity, the theory that applies to the Universe at large scales. However, the radiation that emanates from black holes is made of particles, which fall under the jurisdiction of quantum physics. Unfortunately any naive attempt to combine general relativity and quantum physics in a single mathematical framework is doomed to fail: it leads to irreconcilable contradictions. A unified theory of quantum gravity is the holy grail of modern physics.
Using approaches that had proved successful both in describing the radiation emanating from black holes and in inflationary theory, Hawking has worked on his own approaches to quantum gravity. It is here that the greatest challenges for future generations lie.
"[The challenges] all centre on the question of 'what is the quantum theory of gravity'", says Gibbons. "There are questions that arise about whether the process of evaporation [...] satisfies the laws of quantum mechanics. There are also suggestions that Hawking has made with James Hartle and some of his students about [...] whether quantum mechanics applies to the Universe as a whole. All of these questions are a challenge to constructing a theory of quantum gravity. The work that Stephen did [which Gibbons participated in] is very much a low order approximation: it gives an account to some extent of the quantum mechanics of black holes, but it's incomplete."
Hawking was cautiously optimistic that the search for quantum gravity may be nearing its end. It’s an ambitious hope, but Hawking witnessed changes that would have seemed equally ambitious just a few decades ago. During his lifetime cosmology has grown into a fully-fledged precision science, which has seen spectacular advances and is looking forward to more to come.
Nowhere will this legacy be pursued more eagerly than at Hawking's academic home, the Department of Applied Mathematics and Theoretical Physics at the University of Cambridge, where Hawking built a world-leading research group on general relativity and cosmology. With more observational evidence due to arrive from further analyses of the CMB and from gravitational waves, Hawking leaves us in exciting times and his successors with important ideas to work on.
About this article
Marianne Freiberger is Editor of Plus.
This article first appeared as a feature on the website of the Cambridge Mathematics Faculty.