What is time?
In the latest poll of our Science fiction, science fact project you told us that you wanted to know what time is. Here is an answer, based on an interview with Paul Davies, a theoretical physicist and cosmologist at Arizona State University and Director of BEYOND: Centre for Fundamental Concepts in Science. Click here to see other articles on time and here to listen to our interview with Davies as a podcast.
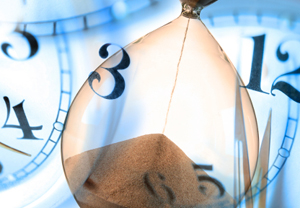
What is time?
Everyone knows what time is. We can practically feel it ticking away, marching on in the same direction with horrifying regularity. Time has enslaved the Western world and become our most precious commodity. Turn it over to the physicists however, and it begins to morph, twist and even crumble away. So what is time exactly?
To many people throughout history time would have been synonymous with the rhythms of nature; the passing of the seasons and the cycles of the celestial bodies. If this idea seems naive today, it's not only because modern clocks are infinitely more accurate time keepers than the celestial bodies ever were. It's also because we've come to think of time as something universal, something that would keep marching on even if all clocks, celestial or man-made, were to stop. The notion of an absolute time, one that's measurable and the same for all observers, was expressed most succinctly by Newton: "absolute, true and mathematical time, of itself, and from its own nature, flows equably without relation to anything external."
Einstein's time
Newton's absolute time may feel like an accurate description of the beast that rules our daily lives, but in science the notion was shattered in 1905 by Einstein's special theory of relativity. "Einstein showed that there isn't a universal time," explains Davies. "Your time and my time get out of step with each other if we move differently." In other words, the duration of time between two events can vary depending on how fast you are moving in the period between the events.
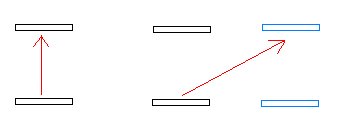
Imagine two observers, one on a train and one stationary. The traveller sends a pulse of light from a torch vertically up. The traveller's view is shown on the left: the pulse travels vertically up. The stationary observer's view is shown on the right: the position of the torch and train ceiling at the start and end of the pulse's journey are shown in black and blue respectively. The pulse travels diagonally.
At the root of this strange time warping effect lies Einstein's postulate that the speed of light should be the same for all observers, no matter how fast they are moving. Imagine two observers, one travelling on a train and the other stationary by the side of the tracks. As the two pass each other the traveller emits a pulse of light from a torch shining vertically up. The two observers will disagree on the distance the pulse has travelled when it hits the ceiling of the train, because the stationary observer perceives not just the vertical motion of the pulse, but also the horizontal motion of the train.
Since both observers measure the same speed of light, and since speed is distance per time, this implies that they must also disagree on the time it took the pulse to travel that distance. Time is relative to the observer, or as the physicist Kip Thorne prefers to put it, time is "personal". (For a more detailed description, read the Plus article What's so special about special relativity?.)
We don't notice this time dilation in daily life, but it's not so small as to be unmeasurable. "If I fly from Phoenix to London and back again, and then compare my clock with that left in the office, they will be out of step with each other by a few billionths of a second," says Davies. That's a tiny amount for humans, but it's well within the measuring capability of modern clocks.
In fact, time dilation has a real impact on the global positioning system (GPS), which many of us have come to rely on for navigating around the world. "The system works with orbiting satellites that are moving very fast," explains Davies. "If you didn't factor in this time distorting effect of motion, then your GPS would very quickly begin to accumulate errors so that in an hour or two you'd be lost. So this is a real effect, not just some sort of mad mathematician's nightmare."
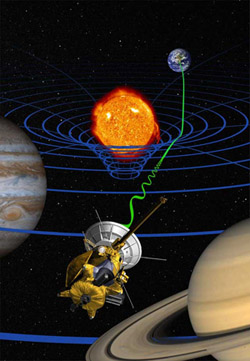
An artist's impression of the Sun warping spacetime and the Cassini space probe testing relativity by measuring how signals are delayed by the warping. Image courtesy NASA.
But motion isn't the only thing that can distort time. In his general theory of relativity, published in 1916, Einstein showed that gravity too can slow time. Rather than thinking of gravity as an invisible force that wafts across the ether, Einstein thought of it as the effect of massive bodies distorting the very fabric of space. A famous analogy is that of a bowling ball sitting on a trampoline, which creates a dip that a nearby marble will roll into. According to general relativity, massive objects like stars and planets warp space in a similar way, and thus "attract" other bodies that pass nearby. However, Einstein realised that time and space are inextricably linked in what he called spacetime, so the warping effect of gravity does not just effect space, but also time.
"Gravity slows time, so that it runs a little bit slower in the basement of your house than it does on the roof," says Davies. "It's a tiny effect, but it can be measured, even on distances that are that small. But if you want a seriously big time warp from gravity, you have to go where there's a very big gravitational field. If you had a clock on the surface of a neutron star, for example, it would tick at about 70% of the rate of a clock on Earth. The ultimate time warp is at the surface of a black hole, where in a sense time stands still relative to our time. If you went there, you wouldn't notice anything peculiar about time, but if you compared clocks between the two locations, they'd be enormously out of step."
Einstein drew an interesting conclusion from his results about the nature of time. In a letter to the family of a recently deceased friend, Michele Besso, Einstein wrote, "... for us physicists believe the separation between past, present, and future is only an illusion, although a convincing one." Since time is relative to the observer, it is impossible to divide it up into past, present and future in a way that is universally meaningful. In some sense, past, present and future are all there at once.
"This notion is sometimes called block time, but I like to call it the timescape because it's a bit like a landscape," says Davies. "If you look at a map, the whole of the landscape is there before you, all at once. If you add time as the fourth dimension on this map, then all of time is there at once too. The fact that nothing in physics singles out a particular 'now' is a mystery."
Incidentally, there is nothing in Einstein's theory that prohibits time travel, be it into the future or into the past. But this is a can of worms we won't open here, as you can read about it in Kip Thorne's Plus article Is time travel allowed? (or read Davies' book How to build a time machine).
The arrow of time
Thinking of past and future brings us to another problem that has foxed scientists and philosophers: why time should have a direction at all. In every day life it's pretty apparent that it does. If you look at a movie that's being played backwards, you know it immediately because most things have a distinct time direction attached to them: an arrow of time. For example, eggs can easily turn into omlettes but not the other way around, and milk and coffee mix in your cup but never separate out again.
The most dramatic example is the history of the entire Universe, which, as scientists believe, started with the Big Bang around thirteen billion years ago and has been continually expanding ever since. When we look at that history, which includes our own, it's pretty clear which way the arrow of time is pointing.
"But the mystery is that the laws of physics show no preference for forward time or backward time," says Davies. For example, if you can make an object move one way by applying a force, then, as Newton's second law of motion tells you, you can make it retrace its path by applying the same force in the opposite direction. So when you watch a movie of this process you wouldn't be able to tell if it's being played forwards or backwards, as both are equally possible.
"So the problem is how to account for the asymmetry of time in daily life when the laws that govern all the atoms that make up everything around us are symmetric in time," says Davies. Much has been made of this problem, which affects Einstein's physics just as it did Newton's classical description of the world.
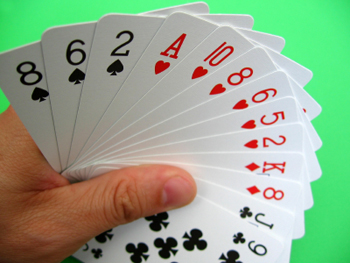
Order or disorder?
But the answer isn't all that difficult to find. Most processes we feel are irreversible in time are those that (for whatever reason) start out in some very special, highly ordered state — Davies uses a pack of cards as an example. When you first open up a new pack the cards will be ordered according to suit and numerical value. When you shuffle them for a while they will become disordered, so it seems that, as time passes, things will always move from order to disorder. "We might think that this is very strange because there is nothing in the act of shuffling that chooses a direction in time, yet we see a distinct arrow," says Davies.
However, there is nothing in the laws of physics that prevents the act of shuffling from producing a perfectly ordered set of cards. It's just that the ordered state is only one of a total of around 8 × 1067 possible states, so the chance that we come across it while shuffling the cards is vanishingly small. So small that it would never happen even within several lifetimes of shuffling.
So the apparent asymmetry of time is really just an asymmetry of chance. Systems of many components — like a cup full of milk and coffee particles or a bowl full of egg particles — evolve from order to disorder not because the reverse is impossible, but because it's highly unlikely. This, in a nutshell, is the second law of thermodynamics, which states that the entropy (a measure of the disorder) in a closed physical system never decreases. It's a statistical principle, rather than a fundamental law describing the behaviour of individual atoms. The apparent arrow of time emerges as a property of the macroscopic system, but it's not there in the laws that govern the individual particle interactions. As the physicist John Wheeler put it, "If you ask an atom about the arrow of time, it will laugh in your face."
This also applies to the whole Universe. "The Universe started out very smooth and expanding uniformly," says Davies. From a gravitational view point the Big Bang was a low entropy state and the Universe has been increasing its entropy ever since, hence the arrow of time. The question now is why the Universe started in the way it did. "Why our Universe went bang in such an ordered state is still a mystery," says Davies. "There is no agreed answer to that, partly because there is no agreed model of cosmology. We all think the Universe began with a Big Bang and we know it's expanding. What we don't know is if the Big Bang is the ultimate origin of time or whether there was a time before that." (Read the Plus article What happened before the Big Bang? for more on this subject.)
Time disappears
What would you like to know next?
This article is part of the Science fiction, science fact project, which lets you vote for questions to put to experts. Vote for the question you'd like us to ask next!
One thing we have neglected to say so far is that Einstein's theory, which describes the macroscopic world so admirably well, doesn't work for the microscopic world. To describe the world at atomic and subatomic scales, we need to turn to quantum mechanics, a theory that's fundamentally different from Einstein's. Reconciling the two, creating a theory of quantum gravity, is the holy grail of modern physics.
When Schrödinger and Heisenberg formulated quantum mechanics in the 1920s, they ignored Einstein's work and treated time in Newton's spirit, as an absolute that is ticking away in the background. This already gives us a clue as to why the two theories might be so hard to reconcile. The status of time in quantum mechanics has also created profound problems within the theory itself and has lead to "decades of muddle and subtlety," as Davies puts it.
We won't go into this muddle here, but we'll note the conundrum that unfolds when you try to apply quantum mechanics to the Universe as a whole (a rather controversial approach not all physicists agree with). "If you try to write down a quantum mechanical description of the whole Universe, you find that the time parameter actually drops out [of the equations], it's not there at all," says Davies. Time is replaced by correlations. "For example, you might have a correlation between the size of the Universe and the value of some [physical] field. We would describe this by saying 'as the Universe evolves over time and gets bigger, so this field changes in value'. We use that language, but actually all you're talking about is a correlation [between physical quantities] and time can be removed completely."
Some people have interpreted this to say that time doesn't exist at all, but Davies disagrees. "I think time exists just as telephones do. It's a real thing and we can measure it. But it does suggest that the way it enters into our description of the world is different from other quantities we're used to."
One possibility is that time, and also space, are emergent properties of the Universe, which are not part of the bottom level of reality. "It may be that for the extreme conditions at the Big Bang a description in terms of other variables is more appropriate. When we see the world with a well-defined space and time [or spacetime as Einstein put it] this may just be some particular state of the Universe that has emerged out of the Big Bang." Davies uses a block of rubber as an example: it's got its very own physical properties, its elasticity for example, but these properties aren't there at the atomic level. They are a result of the atoms and the laws that govern them combining in one particular way. Similarly, the Universe, as it cooled down from the Big Bang, may have just happened to give rise to spacetime. Perhaps, if it had cooled down in another way, spacetime wouldn't have come up.
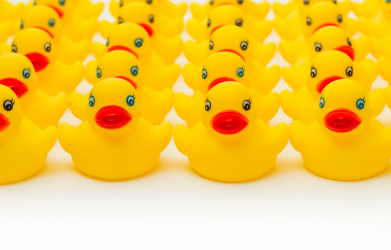
Rubber ducks have emergent properties.
But if space and time aren't fundamental, what are the fundamental properties of the Universe? There is no theory that people agree on. "We can invent words to describe them and people have, but these things are not anything we are going to encounter in daily life. So we're just resorting to [mathematical descriptions]. But even if one day we manage to explain time and space in terms of something else, that only pushes the question to another level, because you then have to explain [the something else]."
So it seems that we're no closer to understanding what time is than Newton was — perhaps we understand it even less. But then, perhaps the job of the scientist isn't to fully explain the Universe, but merely to describe it. "You postulate a theory, usually in the form of mathematical equations, and then you test it against reality," says Davies. "If it does work, you don't argue where those equations come from. It's just your best attempt to describe the world."
Whether it's fundamental, emergent, or just a set of correlations in disguise, the fact is that something we call time manifests itself undeniably and we all know about it. As a friend of mine put it, "If you want to know what time is, just look at my face."
About this article
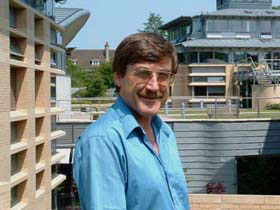
Paul Davies
Paul Davies is a theoretical physicist and cosmologist at Arizona State University and Director of BEYOND: Centre for Fundamental Concepts in Science. He has worked in a number of different fields, including cancer research and astrobiology (Plus has interviewed him on astrobiology in the past) and has written a large number of popular science books, including About time: Einstein's unfinished revolution and How to build a time machine.
Marianne Freiberger, co-editor of Plus, interviewed Davies in July 2011.