Over the last two decades physicists have been developing a curious idea. Perhaps the world we inhabit, including ourselves, is nothing more than an illusion, a hologram conjured up by a reality which lacks a crucial feature of the world as we perceive it: the third dimension.
Juan Maldacena, professor at the Institute for Advanced Study in Princeton, has played a vital role in the development of this idea, which is known as the holographic principle or the AdS/CFT correspondence. In the 1990s Maldacena came up with the very first model of a universe which realises the holographic principle. He explained us his ideas on a visit to Cambridge in 2009. This article is a shortened version of a longer article we wrote at the time.
The holy grail of modern physics
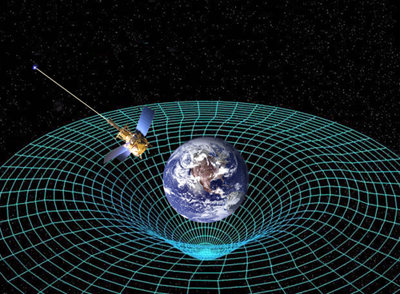
According to general relativity, massive bodies warp spacetime. Image courtesy NASA.
The holographic principle grew out one of the biggest scientific problems of the twentieth century: the fact that the two fundamental theories of physics, Einstein's theory of gravity (general relativity) and quantum mechanics, do not get along with each other.
While general relativity describes the world of planets and galaxies, quantum mechanics looks at the sub-atomic scale, the realm of the fundamental particles that make up matter. At these small scales, there's little mass and gravity is negligible. To make things consistent, it would be nice to re-write Einstein's theory of gravity in the same terms as quantum field theory, the highly successful quantum mechanical description of particle physics. However, any naive attempt to do so fails. "A naive quantisation of gravity doesn't work and leads to mathematical inconsistencies," said Maldacena. "We need something new."
One strong contender for a theory of quantum gravity is string theory, which gets around the mathematical problems of a naive quantum gravity. It's a mathematical theory whose elegance has captured the hearts of many physicists. The downsides are that string theory does not as yet give a complete description of the world and that it has not, and currently cannot, be tested. Nevertheless, it is string theory which handed Maldacena a clue to solving the quantum gravity riddle: by turning gravity into an illusion conjured up by a quantum hologram.
Black holograms
The conflict between general relativity and quantum mechanics poses no problem for most practical purposes, as physicists usually look at either the large-scale world, where quantum effects do not come into play, or at the small-scale world, where particles are light and gravity has little effect. But there is one situation in which the clash of the two theories is tangible: black holes are formed when a large amount of mass is concentrated in a tiny region of space. The resulting gravitational pull is so strong that nothing can escape from a black hole, not even light, so there's no way you can ignore gravity when thinking about black holes. The small scale means that quantum effects also come into play. To describe what's going on in a black hole, you really do need a unified theory of quantum gravity.
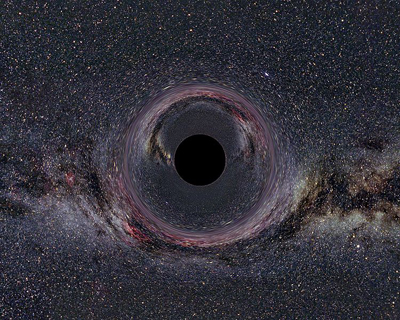
A simulated black hole with the Milky Way in the background. Image courtesy Ute Kraus, Space Time Travel.
Black holes are also what first gave rise to the holographic principle. Theoretical advances in their understanding, mostly developed by Stephen Hawking and Jacob Bekenstein in the 1970s, caused the physicists Gerardus 't Hooft and Leonard Susskind to consider how much information you can squeeze into a region of space (you can find out more in this article).
Information manifests physically, whether it's in the pages of a book, the neurons in your brain, or the photons that whiz up and down fibreoptic cables transmitting information over the Internet. This physical manifestation involves energy. And since energy is equivalent to mass (recall Einstein's E=mc2), squeezing information into a confined region of space is akin to squeezing mass into it. If you try and squeeze too much mass/information into it, you form a black hole, so there's a limit to the information content of a confined region of space that's not a black hole. Such a region comes with a boundary which, like the shell of an egg, is a two-dimensional surface whose area you can measure. When 'T Hooft and Susskind calculated the limit of the information content of a region of space, they found that it is measured by the surface area of the region's boundary.
"This sounds like something very simple and innocent," said Maldacena, "but in all other descriptions we have of the world, the number of variables grows like the volume." For example, the number of apples you can fit into a box is proportional to the volume of the box, not to the surface area of its walls. According to intuition, information should also grow in line with volume, and not, as the holographic principle suggests, with surface area. If the holographic principle is true, then our three-dimensional approach to physics overshoots the mark. We should be able to get by with a leaner version, one that hinges on area, rather than volume. This raises the mind-numbing question of whether the third dimension is actually real, or merely an illusion, like the three-dimensional image created in a hologram.
So far, nobody has found a precise formulation of the 2D version of physics that describes our 3D world. However, in 1995 Susskind re-defined string theory with the holographic principle as a central pillar. And in 1997, at the tender age of 29, Juan Maldacena came up with the first ever concrete description of a holographic universe.
Toy physics
Maldacena's universe is not like the one we actually live in: it's a model, a toy universe, which comes complete with its own physics. It's a hologram because all the physical goings-on inside it can be described by a physical theory that's only defined on the boundary. What's more, it's a universe in which the gravity/quantum conundrum has been resolved completely: the boundary theory is purely quantum, it contains no gravity, but a being living in the interior will still experience gravity. Gravity in this universe is part of the holographic illusion.
The toy universe is known as anti de Sitter space, after the Dutch physicist Willem de Sitter. You can find out more about it in the longer version of this article. Anti de Sitter space is very different from the world we actually live in — both space and time are distorted in strange ways — but this shouldn't stop you from inventing some physics for it. All you need is a notion of the fundamental objects of this physics — fundamental particles and forces — and some mathematical laws describing their interaction. Maldacena used a version of string theory to describe the physics that happens in the interior of his model universe. As you'll recall, string theory includes both gravity and quantum mechanics, so a being living inside Maldacena's world will experience gravity in a similar way as we do.
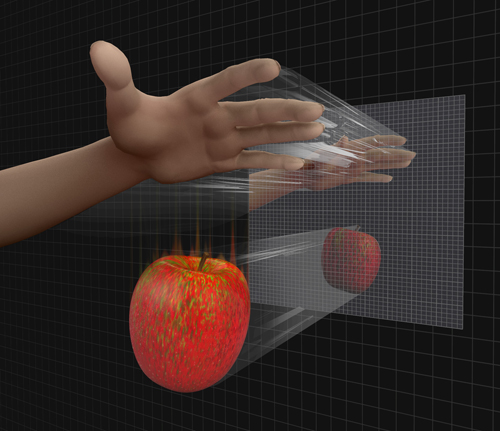
A holographic Universe.
Maldacena's crucial observation was that the string theory describing the interior of his universe has a sort of "shadow" on the boundary of the universe: you can define a quantum field theory on the boundary so that every fundamental particle in the interior has its counterpart on the boundary, and so that every interaction between interior particles corresponds exactly to an interaction between boundary particles. You can now describe the action of, say, dropping an apple in the interior purely in terms of the boundary theory. You can even choose to ignore the interior altogether without losing any information at all — this world is a true hologram.
The important point from a quantum gravity angle is that the boundary theory is a well-understood quantum theory of particle physics, very similar to the one we use to describe sub-atomic processes in nature. Referring only to small scales, it doesn't bother with gravity. Yet it is able to describe the esoteric quantum gravity theory which governs the interior. It's the first ever complete description of a quantum spacetime.
But what does it mean for us?
Maldacena's model is just that, a model. We do not yet know if the universe we live in is a hologram, and we still don't have a consistent quantum description of gravity that applies to our world.
But what if it turns out that the holographic principle does apply to the world we live in? Will this mean that we, along with spacetime, are just an illusion? "Yes, you could say [we are] an illusion, or an emergent phenomenon," said Maldacena. "If we lived in such a universe we would be, in some sense, approximate descriptions. But that's nothing new in physics. Take the surface of a lake, for example. It seems to be a well-defined surface, insects can walk on it. But if you look with a sufficiently powerful microscope, you'll see that there are molecules moving around and there is no sharply defined surface. The idea is that spacetime could be similar. It's not well-defined in an absolute sense, but we are so big that we don't notice it." Just like the insects on the lake, we'd be looking at the world with eyes that are too crude to reveal the true nature of spacetime. Ignorance is bliss, so in an every day practical sense, whether or not we live in a hologram probably doesn't really matter — though there's endless fun to be had with the philosophical side of things.
But what about Maldacena himself? Does he really believe that the holographic principle is true? "Well, I view this idea as a model, but it's a model that gives a mathematical description of quantum spacetime. So we should take it seriously until someone refutes it, or comes up with something better."
About this article
Juan Maldacena is a Professor at the Institute for Advanced Study in Princeton. He was interviewed by Marianne Freiberger, Editor of Plus, in Cambridge in April 2009. This is a shortened version of a longer article, published at the time.
This article now forms part of our collaboration with the Isaac Newton Institute for Mathematical Sciences (INI) – you can find all the content from the collaboration here.
The INI is an international research centre and our neighbour here on the University of Cambridge's maths campus. It attracts leading mathematical scientists from all over the world, and is open to all. Visit www.newton.ac.uk to find out more.
