
Brief summary
Physicists have explored whether it is possible to detect hypothetical boson stars via the gravitational waves their collisions would emit. Since boson stars are candidates for the so-called dark matter that exists in the Universe but whose make up we don't understand, a detection of a boson star would count as a major breakthrough towards our understanding of dark matter.
Physicists like nothing better than discovering new phenomena that conflict with current theories. Now a team at the University of Cambridge have delivered a new approach to doing just that — which may also help to solve one of cosmology's great mysteries.
Ripples through space and time
In 2015 the world of physics experienced a revolution. For the first time ever physicists observed a tell-tale signal from a gravitational wave — a ripple in the very fabric of spacetime that had been caused by two black holes colliding over 1 billion light years away. The existence of gravitational waves had been predicted as a consequence of Einstein's theory of gravity, the general theory of relativity, but it took a measuring instrument of extremely high precision, the Laser Interferometer Gravitational-wave Observatory (LIGO), to detect one.
Since then around 100 gravitational wave signals have been observed. Most of them emanate from colliding black holes but a handful of collisions involve neutron stars.
The reason these events cause spacetime ripples is that black holes and neutron stars are extremely dense. According to general relativity, massive objects cause the fabric of spacetime to curve — the smaller the region the mass is concentrated in (the denser the object) the higher the curvature. If two objects of extremely high density begin spiralling around each other and then collide, the effect on spacetime is strong enough to send ripples travelling through the Universe.
If you have a hammer everything looks like a nail
Gravitational waves have provided wonderful new windows on the Universe: by detecting them we can learn about the objects and processes that cause them. At the same time gravitational wave physics has been caught in a kind of trap. The only things physicists knew how to observe using gravitational waves were black holes and neutron star mergers. They simply didn't know what anything else would look like — and for this reason the only thing they have in fact observed were black hole and neutron star mergers.
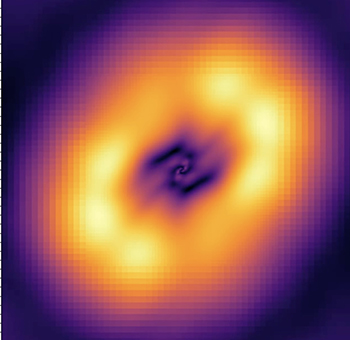
A snapshot from the team's numerical simulations showing two boson stars merging. Image by Tamara Evstafyeva using the GRChombo code as part of the GRTLCollaboration.
"It's a bit like finding a blurred fingerprint at a crime scene and checking it against your database of known fingerprints," says Ulrich Sperhake, Professor of Theoretical Physics and member of the Stephen Hawking Centre for Theoretical Cosmology at the Department of Applied Mathematics and Theoretical Physics (DAMTP) at the University of Cambridge. "If you find a good match in the database you'll go after the person it belongs to. But what if the perpetrator is a chimpanzee? Since you don't have a database of chimpanzee fingerprints, you have no chance of catching the real culprit. And you might even mistake them for an innocent human with a similar fingerprint."
The new result addresses this problem. Authors Tamara Evstafyeva, Ulrich Sperhake, Isobel M. Romero-Shaw and Michalis Agathos, set out to identify fingerprints of a different species than black holes and neutron stars that could be detected in gravitational wave signals. They involve collisions of stars of a kind we don't know exists but whose detection would herald new physics: boson stars.
Boson stars and dark matter
The fundamental particles of nature come in two groups. There are fermions, which include familiar particles such as electrons and protons, and there are bosons. Examples of bosons are photons, which are particles of light, and the famous Higgs boson which was experimentally observed for the first time back in 2012.
The stars that we know of, our Sun, neutron stars, and white dwarves, for example, are made of fermions. Boson stars would be made entirely of bosons. People started wondering whether such stars could exist because the only bosons that have ever been caught in the wild were caught as individual particles or in small bundles — it would be interesting to know whether they're capable of forming macroscopic structures such as stars.
The bosons that make up a boson star would have to be fundamentally different from the bosons we know of, however. In particular, they would likely not interact with light which means that we wouldn't be able to see them. And this is where some of the excitement lies: physicists have known for some time that there must be more matter in the Universe than we can see because of the gravitational pull that this so-called dark matter exerts on visible objects. Exactly what this dark matter is made of is one of the biggest open questions in physics today.
"There are lots of ideas to explain dark matter in terms of the kind of bosons that could also make up a boson star and this is where our work comes into play," says Sperhake. "If we detect a boson star we have an observation of a serious dark matter candidate."
Luckily in terms of gravitational wave signals, boson stars could also be extremely massive and dense so their collisions and mergers should generate gravitational waves. The key question Sperhake and his colleagues set out to answer is whether we would actually be able to observe these waves and tell them apart from those generated by black hole and neutron star mergers. Even if the objects generating these signals came from something other than the boson stars as the theory envisages them, a detection would be sensational. "We regard boson stars as a proxy for anything else that is not really a black hole or neutron star — we are looking for new physics."
Simulating signals
To answer their question the team used all the theoretical insight we have into hypothetical boson stars to simulate, on a computer, the gravitational wave signals that a merger of two boson stars would produce. They considered special instances of two types of merger: one where the two boson stars merge together to form another boson star, and one where the merger results in a black hole. The simulations themselves count as a major achievement. "These are what we regard as the first numerical simulations of boson stars that are of a quality high enough to be used in gravitational-wave data analysis," says Sperhake.
The team then subjected the simulated gravitational wave signal to the statistical techniques that physicists currently use to dig a clear signal out from all the noise a gravitational wave detector such as LIGO observes, and to classify the objects that caused the signal. "We wanted to ask, if a hypothetical boson star merger were to happen in the Universe, what would current gravitational wave data analysis techniques tell us about it," says Evstafyeva, who was Sperhake's PhD student at the time the work was conducted and has now moved on to the Perimeter Institute for Theoretical Physics. "Would it classify it as something that is not a black hole, or would it perhaps mistake it for a black hole?"
Results showed that in the case when the hypothetical boson star merger results in another boson star, the data analysis would clearly show that new physics is present in the data. "In this case the gravitational wave signatures have a smoking gun effect — they look very different from a black hole merger," says Evstafyeva. "If we were to detect such signals and they were loud enough, then we would definitely be able to conclude that this is not a black hole, but something new."
One of the team's simulations showing two boson stars colliding and merging to form a new boson star. In this case analysis showed that the gravitational wave signal would be distinguishable from a black hole merger. Movie by Tamara Evstafyeva using the GRChombo code as part of the GRTLCollaboration.
In the case where the boson star merger results in another black hole, results were at first glance a little disappointing. "In this case the signal would currently be mistaken for a signal coming from a black hole merger," says Evstafyeva.
There is hope for the future, however. The team have shown that although signals from boson star mergers can be very similar to those from black hole mergers, the two are not identical. Next generation gravitational wave detectors, hoped to come online in around ten years' time, will pick up less noise than current ones. With additional statistical tests it may then be possible to detect the difference between the two types of signal. "There is a decent amount of hope that eventually we will be able to distinguish between them," says Sperhake. In the meantime the researchers will keep building their database of boson star fingerprints for detectors to look out for.
One of the team's simulations showing two boson stars colliding and merging to form a black hole. In this case analysis showed that the gravitational wave signal would currently not be distinguishable from a black hole merger. Movie by Tamara Evstafyeva using the GRChombo code as part of the GRTLCollaboration
If a new type of matter were to be discovered through an observation of boson stars, then this would modify our understanding of particle physics — adding the particles that make up the new matter to the family of particles we already know. But the discovery of new physics may also mean that general relativity itself may have to be modified to make room for new phenomena. The team have been investigating the new results in this light too.
"This will definitely make the 'testing general relativity' community very happy," says Evstafyeva. "They have been looking for deviations from vanilla gravitational wave signals for quite a while now."
About this article
Tamara Evstafyeva is Vera Florence Cooper Rubin Post Doctoral Research Fellow at the Perimeter Institute for Theoretical Physics. Prior to that she completed her PhD at University of Cambridge. Her research interests are in gravitational wave physics, numerical relativity and data analysis
Ulrich Sperhake is Professor at DAMTP, University of Cambridge. His research interests are the exploration of extreme gravity physics, black holes and other compact objects.
Marianne Freiberger, Editor of Plus, interviewed Evstafyeva and Sprhake in October 2024.